In 1882, Thomas Edison flipped on the switch at the Pearl Street Station, the world’s first permanent power plant. Eighty customers within a one-kilometer radius formed this earliest instance of “the grid.” This model proved highly effective, with business expanding to over 500 customers within two years. But other power stations soon got in the game, and the edges of these small grids eventually started to touch each other. Soon, the power industry began to transition from small local grids to the larger interconnected grid that we’re familiar with today.
But over the last few decades, researchers, developers, and communities have been exploring a return to the grid’s small beginnings with the rise of microgrids. In the first post in this series, we started with the example of a single home with a solar + storage system. We classified this system as a distributed energy resource (DER) and defined the context in which it fits—a historically centralized grid that is rapidly changing. This post will explore how the grid developed, and why recent developments are moving back to smaller grids: microgrids.
Building a standardized grid
When smaller grids like Edison’s began, each used different electrical systems, which soon resulted in overlapping, incompatible coverage. Eventually the industry settled on a convention utilizing three innovations: alternating current (AC), transformers, and three-phase power.
In an AC power system, as the name suggests, the direction of current flow alternates back and forth, as opposed to only flowing one direction in a direct current (DC) system. AC power has the unique benefit of being easily converted (or “stepped”) to higher voltages using a transformer, a simple device consisting of coils of wire around a thick steel ring. At higher voltages there is less resistance to the flow of electricity, so AC power can be transmitted long-distances efficiently. At homes and businesses, additional transformers step power down to lower voltages that are easier to use and reduce the risk of injury from electric shock.
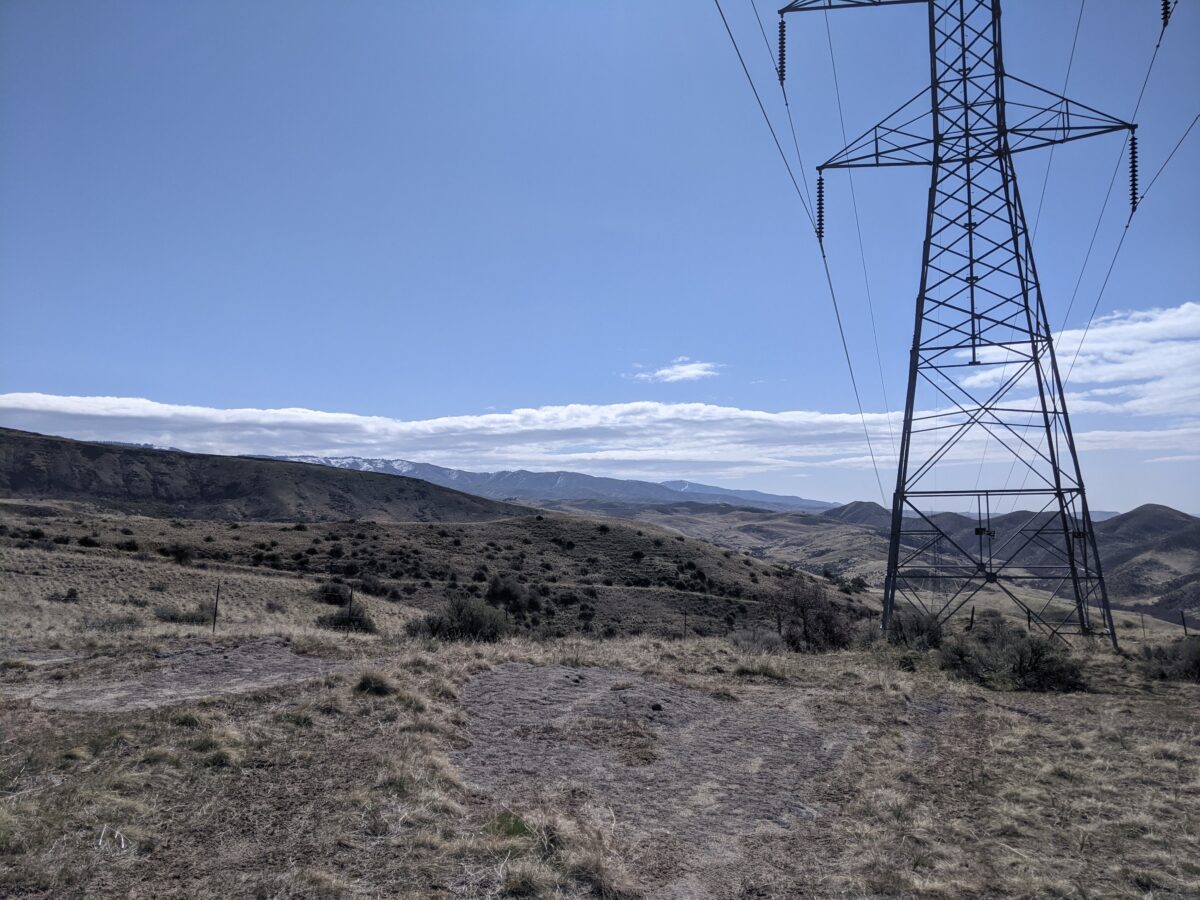
A three phase system is the combination of three AC systems (“phases”). This arrangement enables constant power transfer, since current will only be crossing zero (switching directions) in one phase at a time. Two phase systems also have this feature, but in a three phase system, the three currents always balance each other, allowing more power to be transmitted on the same size wires.
Back to the beginning
This basic approach—three-phase AC power—has persisted over the last hundred years, becoming the basis of what’s been called the world’s largest machine, or today’s interconnected power grid. But with the advent of cheap, reliable distributed energy resources, an opportunity exists to revisit the hyper-local origins of the grid.
In 2002, researchers at the University of Wisconsin-Madison were the first to coin the term “microgrid,” referring to a group of energy sources and loads and the control system to allow it to operate with or without the larger power grid. The US Department of Energy provides the following formal definition:
A microgrid is a group of interconnected loads and distributed energy resources within clearly defined electrical boundaries that acts as a single controllable entity with respect to the grid. A microgrid can connect and disconnect from the grid to enable it to operate in both grid-connected or island-mode.
The second half of this definition encapsulates the key value of microgrids—they can operate alongside the grid or even create their own separate electrical “islands.” In simple terms, a microgrid is a portion of the distribution grid with its own power sources that can connect and disconnect from the grid.
For buildings connected to the microgrid, this enables all of the benefits of conventional DERs, such as lower emissions and reduced utility bills, along with the resilience and reliability benefits of a backup power source.
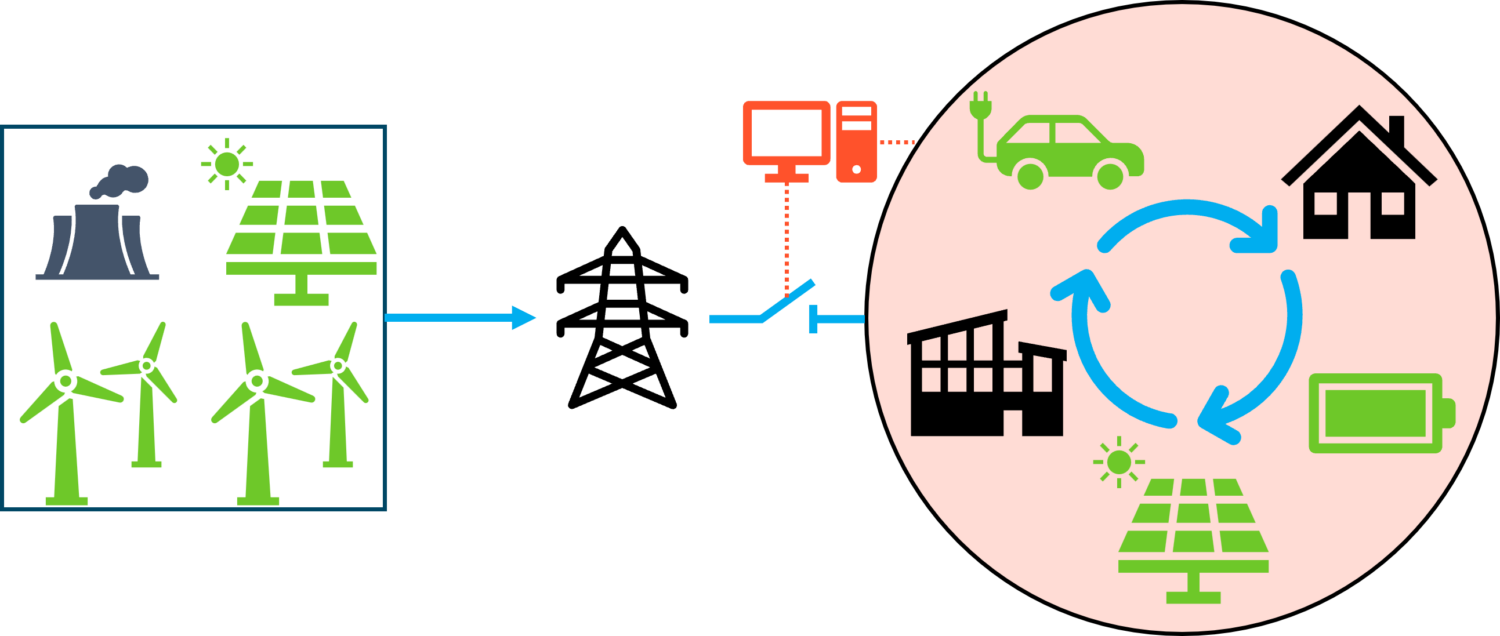
This distinction is important because one of the most prevalent DERs—solar photovoltaic (PV)—typically can only generate power when there’s an active grid connection. While there are both technical and safety reasons why this makes sense most of the time, it means that solar PV cannot provide energy when it is most needed: during a power outage. But when configured as part of a microgrid, solar PV and other DERs can be used to provide reliable power, regardless of the grid status.
Microgrids in the real world
Microgrids can vary greatly in size and complexity: some serve a single building, while others serve entire communities. Fire Station 1 in Portland, Oregon, is an example of a single-building microgrid. While the building has had a diesel generator for backup power since it was established, recently the City of Portland added solar PV, batteries, and a microgrid controller to reduce the use of diesel, save on costs, and minimize emissions. The city is also using the equipment to train first responders on how to deal with emergencies involving DERs.
Some microgrids consist of literal islands, like the one on Kodiak Island, Alaska, where a microgrid integrating wind, storage, and hydropower enabled the island to drastically reduce diesel consumption, increasing renewable generation from 80% to nearly 100%. In addition to a cleaner power supply, a key outcome of this microgrid—and a motivating factor for its installation—was cost savings: utility rates for residents after the microgrid was operational in 2015 were lower than rates in 2001.
Remote microgrids can also help serve other needs besides resilience and cost-savings. In California, utility PG&E is deploying 12 microgrids in remote regions of its service territory to enable long transmission lines through wildfire-prone areas to be decommissioned. Removing these lines both reduces the risk that these lines would spark wildfires and provides resilience for the communities they serve when lines are damaged by fires or shut off to reduce fire risk.
Barriers to building microgrids
While each of the examples above demonstrate their clear benefits, there are challenges to establishing microgrids. The first barrier is cost—NREL estimates that the infrastructure needed to establish a microgrid can add up to 38% to the total cost beyond just the cost of the DERs themselves.
While single-building and isolated microgrids are straightforward, larger, grid-connected microgrids can run into several challenges. On the technology side, as microgrids get larger, coordinating multiple energy sources can become challenging. Dedicated, high-speed communications networks connecting multiple sources and loads together is an effective but very costly approach with reliability and cybersecurity concerns. Recent research from NREL has explored emulating some of the simpler approaches used to manage the grid that rely on the physics of how electricity works. On the policy side, there are challenges to establishing microgrids with multiple users (such as community-scale microgrids) due to how most states define and regulate utilities. Despite being a leader in many ways in microgrid development, California recently finalized regulations making it practically impossible for anyone but a utility to implement a community microgrid. This means communities in need of microgrids are forced to work with their utility, a sticking point when utilities are often responsible for the conditions that make communities consider microgrids in the first place.
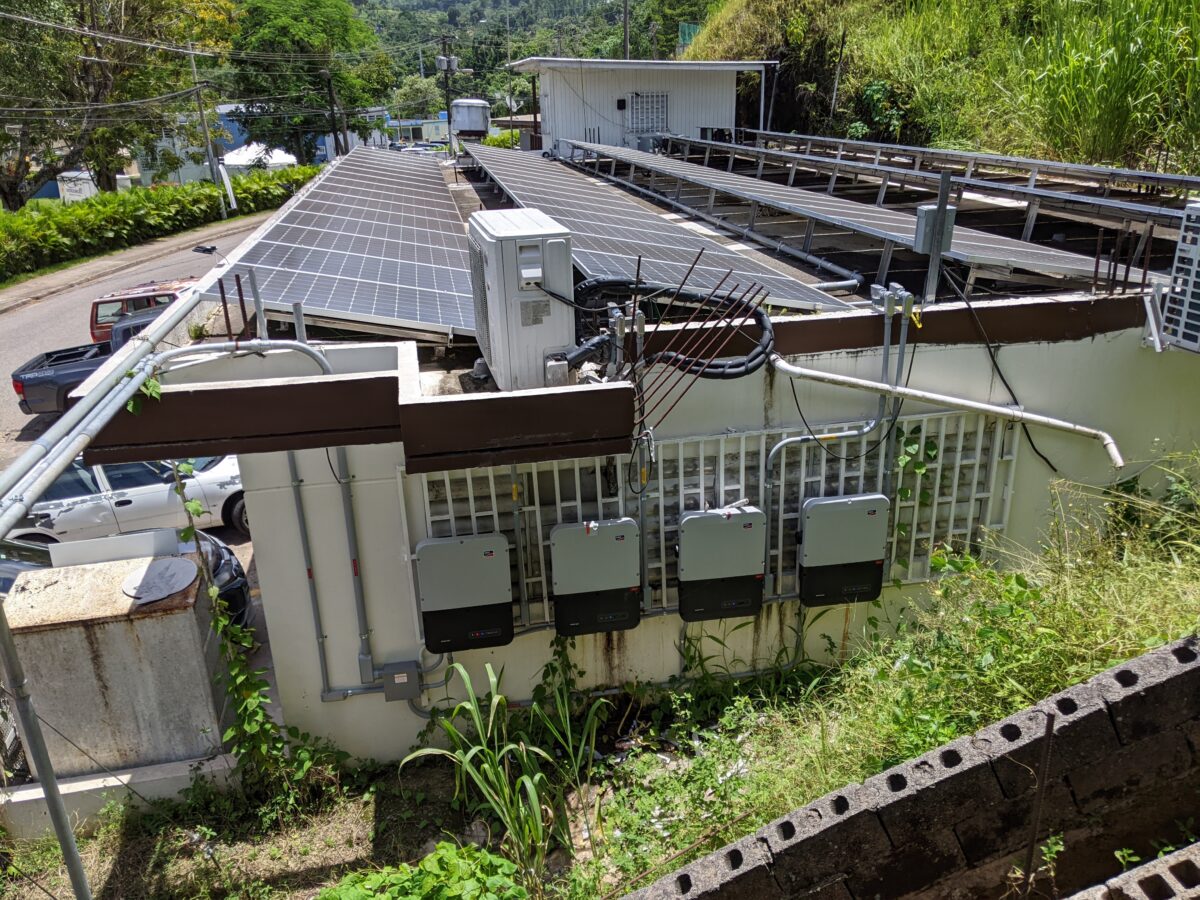
As I learned during a site visit, the only way that residents of Castañer, Puerto Rico, were able to establish their multi-user microgrid was by creating a bespoke process with the utility involving redundant switches and metering, and solar arrays that are effectively shut off for long stretches of the day. (The final post in this series will explore these policy and regulatory barriers further.)
But there is also some progress being made. Last year, Maine updated its utility regulation allowing microgrids to operate electrical infrastructure in rights-of-way just like conventional electric utilities. And in Michigan, UCS and our partners have been involved in efforts to enact similar changes.
Toward a more connected future
Microgrids represent a modern evolution of the grid’s earliest form: local, self-reliant, but now smarter and cleaner. As more states adopt policies making it easier to establish microgrids, communities will increasingly be able to take advantage of them to meet goals around resilience, cost-savings, pollution reduction, and energy independence. While the strength of a microgrid is its ability to operate independently from the grid, it does this by tightly coordinating multiple systems, including energy sources and loads. In the next part of this series, we’ll look at how virtual power plants—systems which also coordinate multiple resources—can bring many of these same benefits without a microgrid’s need to be physically connected together.